
Previous Research Projects
-
Our models allow us to make educated guesses about how different peptide sequences' charges will change as a function of pH.
-
We quantitatively analysed the charge regulation in various peptides as model polyampholytes by combining coarse-grained simulations with various experimental methods.
-
Simulation infromations:
-
Model: the coarse-grained model of a flexible polymer
-
Software: ESPResSo simulation software that we used to simulate the CG model.
-
The constant-pH ensemble accounts for the ionisation reactions of acid and base groups.
-
​
Please see Lunkad et al. (2021a), Lunkad et al. (2021b) for more details.
​
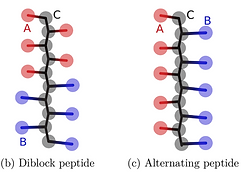
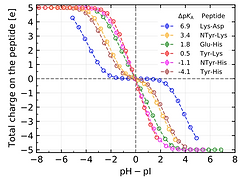

-
The mechanism of protein–polyelectrolyte complexation on the wrong side of the isoelectric point has long puzzled researchers.
-
To understand this mechanism, we simulated a CG model of short peptides containing acidic and basic residues arranged in a blocklike or alternating sequence with star-like polyelectrolytes.
-
Our simulations of these peptides, interacting with polyelectrolytes, showed that charge patch and charge regulation alone can both lead to adsorption on the wrong side of the pI value.
-
We also developed a general framework for assessing the role of the charge-patchiness (CP) and the charge-regulation (CR) mechanisms in existing case studies and for predicting how various proteins interact with polyelectrolytes at different pH values.
-
Simulation infromations:
-
Model: the coarse-grained model of a flexible polymer
-
Software: ESPResSo simulation software that we used to simulate the CG model.
-
The constant-pH ensemble accounts for the ionisation reactions of acid and base groups.
-
​
Please see Lunkad et al. (2022) for more details.

The role of charge-patchiness:



The role of charge-regulation:



-
Examine how the charges of various polyzwitterions are affected by changing the pH of a solution.
-
Our models allow us to make educated guesses about how different polyzwitterions charge will change as a function of pH.
-
By comparing various experiments with theoretical predictions, we could show that molecular simulations can predict and explain the origin of the differences between the effective and bare pKa values of individual titratable groups.
-
Simulation infromations:
-
-
Model: the coarse-grained model of a flexible polymer
-
Software: ESPResSo simulation software that we used to simulate the CG model.
-
The constant-pH ensemble accounts for the ionization reactions of acid and base groups.
-
​
Please see Lunkad et al. (2022) for more details.
​
​
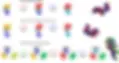

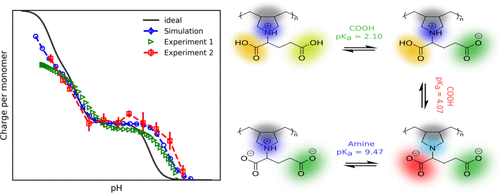
-
We developed a new method for coarse-grained simulations of acid−base equilibria in a system coupled to a reservoir at a given pH.
-
This method can be used for simulations of any reactive system coupled to a reservoir of known composition.
-
the Grand-reaction method accounts for both effects (the Donnan effect due to the partitioning of ions and the polyelectrolyte effect due to electrostatic repulsion along the chain ) on the results and allows us to quantify them.
-
This method can be applied to simulate systems with weak polyelectrolyte gels in a reservoir or attached to a surface, interpolyelectrolyte
complexes in equilibrium with a reservoir solution, or peptides or proteins in salt solutions. -
Simulation infromations:
-
-
Model: the coarse-grained model of a flexible polymer
-
Software: ESPResSo simulation software that we used to simulate the CG model.
-
The constant-pH ensemble accounts for the ionization reactions of acid and base groups.
-
The reaction ensemble algorithm (RxMC) accounts for chemical reactions taking place in the system and for the exchange of particles between the system and the reservoir.
-
​
Please see Landsgesell et al. (2020) for more details.
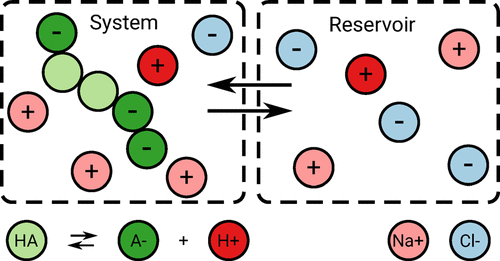
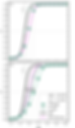
a) Ideal system
b) Real system
-
Surfactants, co-surfactants, and water systems self-assemble into a wide variety of topologically distinct mesoscopic structures.
-
Investigation of the influence of water concentrations on phase transformations of a surfactant/co-surfactant/water system.
-
At higher water concentrations, the surfactant/co-surfactant/water system self-assembles into spherical micelles, which transform into strongly interdigitated one-dimensional rippled lamellar phases upon decreasing water concentrations.
-
Our simulations reveal that the concentrations of water can influence available space around the head groups which couple with critical thickness to accommodate the packing fraction required for respective phases.
-
Simulation infromations:
-
-
Model: All-atom model
-
Software: Gromacs simulation software
-
​
Please see Lunkad et al. (2017) for more details.

